Hand-hygiene to tackle the spread of disease through air transport
- Like
- Digg
- Del
- Tumblr
- VKontakte
- Buffer
- Love This
- Odnoklassniki
- Meneame
- Blogger
- Amazon
- Yahoo Mail
- Gmail
- AOL
- Newsvine
- HackerNews
- Evernote
- MySpace
- Mail.ru
- Viadeo
- Line
- Comments
- Yummly
- SMS
- Viber
- Telegram
- Subscribe
- Skype
- Facebook Messenger
- Kakao
- LiveJournal
- Yammer
- Edgar
- Fintel
- Mix
- Instapaper
- Copy Link
Posted: 7 November 2018 | International Airport Review | No comments yet
A research study aims to identify the effects of hand washing on the global spread of infectious diseases. Preliminary results show that the frequency with which we now embark upon air travel has increased the rate of contagion, but something as simple as hand washing can decrease the chances of a mass spread.
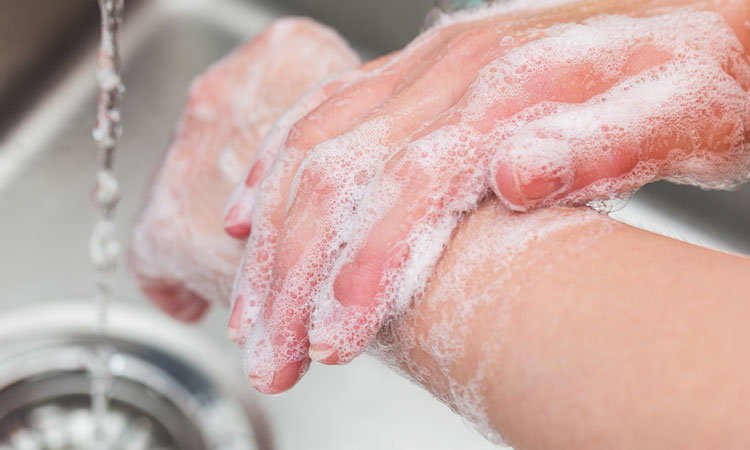
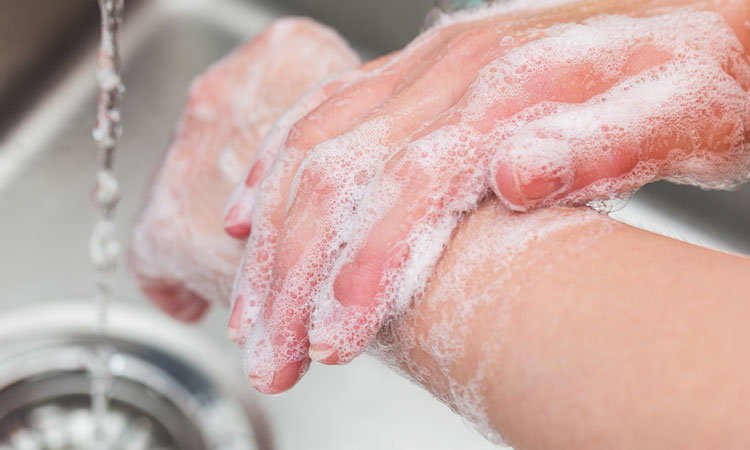
Transmission of bacteria, pathogens and viruses often cause epidemics that spread rapidly in all over the world. The transmission of bacteria is accelerated through physical contact between humans and is strengthened when a dense population is concentrated in confined spaces with lack of proper hygiene and efficient air ventilation. After an outbreak, infectious and bacterial diseases diffuse, when the infected individuals are incorporated into the population transmitting pathogens to susceptible individuals.
Airports play a major role in such transmissions, as they contribute on a daily mixture of people from all over the world, where some of them carrying endemic infections and bacteria from their country of origin. Also, at airports and inside aircraft there are numerous highly contaminated surfaces which are frequently touched by the passengers. Self-service check-in screens, gate bench armrests and water fountain buttons at airports, as well as seats, tray tables and handles of lavatories in aircraft, have high microbial contamination1.
Past epidemics show how infectious diseases spread rapidly around the world through the air transportation network. Examples include the widespread influenza, the severe acute respiratory syndrome (SARS) and several others. SARS initial outbreak occurred on February 2003, where a guest at a hotel in Hong Kong, transmitted an infection to 16 other guests in a single day. These guests seeded outbreaks in Hong Kong, Toronto, Singapore and Vietnam, and within few weeks the disease became an epidemic affecting over 8,000 people in 26 countries across five continents2. For the H1N1 flu, which caused about 300,000 deaths worldwide, the timeline was similar with SARS, as the initial outbreak reported in Veracruz, Mexico on April 2009 and within few days the infection appeared in the U.S. and in Europe, while two months later the WHO and the CDC declared the disease as a pandemic.
The spread of epidemics does not only affect the global public health, but it has also huge socio-economic effects which are not restricted in the countries that are directly affected by the disease. In the case of a pandemic, a massive economic global cost is generated as a result of the reduction of various goods and services consumption, the reduction of tourism and transactions of foreign capitals, and the increase of operating expenditures for several businesses. Even the relatively short-lived SARS epidemic in 2003 which led to the cancellation of numerous flights, the closure of schools and a huge panic in Asian markets, cost the world around $40 billion.
Hypothetical scenarios of global pandemics give estimations on the economic effects. A mild pandemic it is estimated to cost the world 1.4 million lives and a reduction of the total output by nearly one per cent or approximately $330 billion during the first year. In a worsen pandemic scenario, a massive global economic slowdown is expected to occur, with more than 142 million deaths and a shrinking of developing countries’ economies by half. The loss in output in this scenario could reach $4.4 trillion, 12.6 per cent of global GDP in the first year. In the most severe scenario where markets shut down entirely, cost shocks play a much larger role in the GDP losses.
Until today, there is no absolute effective method for preventing the spread of a disease during an outbreak, epidemic, or pandemic as the effectiveness of a prevention is specific on the severity, virulence and other characteristics of the disease. Even vaccination is not sufficient as requires experiments, time and money. On the other hand, hand-hygiene is considered by both CDC and WHO as the most effective and cost-efficient prevention mechanism against a potential pandemic. Frequent and effective hand washing with water and soap significantly reduces the number of bacteria on hands, while hand sanitisers do not have the expected results3.
While hand-hygiene is the most effective mechanism, the capacity of hand washing facilities in public areas is limited only to wash basins at washrooms. Scientific research shows that hand washing with soap prevents disease in a more straightforward and cost-effective way than any single vaccine or medical treatment4. In addition, the wider public is not aware of the impact of hand-hygiene against a global disease propagation. New “smart” technologies aim to increase the capacity of facilities even outside washrooms, to enhance the solutions for room and surface sterilisation and to aware and boost air travellers to wash their hands more effective and frequently. Examples of smart technologies exploration include an antimicrobial system from Airbus that, when is injected on to surfaces will eliminate most of the viruses and pathogens5, a prototype self-sanitising lavatory from Boeing, that will kill 99.99 per cent of pathogens using ultraviolet light6, autonomous robotic systems for dirt detection and cleaning of contaminated surfaces7 and touch-free hand washing stations from Smixin that are able to kill at least 95 per cent of germs and viruses8 in seconds while using 90 per cent less water and 60 per cent less soap than conventional methods.
Smixin AG is currently funding a scientific research conducted by researchers at University of Cyprus (UCY), the Massachusetts Institute of Technology (MIT) and the University of California Berkeley (UC Berkeley) to investigate contagion dynamics through the world air transportation network and to analyse the impact of hand-hygiene behavioural changes of air travellers against the spreading of epidemics worldwide. The study uses well-established methodologies9,10, applies simulations to track travelling agents and their hand-washing activity and analyse the expansion of flu-type epidemics through the world air-transportation network. From the simulation results, the early-time spreading power of the major airports in the world under different hand-hygiene scenarios is measured, and effective and cost-efficient strategies of hand-hygiene policy implementations are identified.
The researchers, using data-driven calculations, first conclude that if we were able to go through all the airports in the world and estimate how many people have cleaned hands, we would find out that mostly one out of five people are cleaned at any given moment in time (i.e. 20 per cent of airport population). This is translated to hand washing engagement rate among the non-cleaned individuals equal to 0.12 per hour (i.e. every hour about 12 per cent of the non-cleaned individuals are washing their hands).
Preliminary results reveal that, if we are able to increase the level of hand cleanliness at all airports in the world (3,650 airports) from 20 per cent to 30 per cent (equivalent to increasing the hand washing engagement rate from 0.12 to 0.21 per hour), either by increasing the capacity of hand washing and/or by increasing the awareness among individuals and/or by giving the right incentives to individuals, a potential infectious disease will have a worldwide impact that is about 21.2 per cent smaller compared to the impact that the same disease would have with the 20 per cent level of hand-cleanliness (or 0.12 per hour hand washing engagement rate). Increasing the level of hand-cleanliness to 60 per cent (or equivalently the hand washing engagement rate among non-cleaned individuals to 0.73 per hour) at all airports in the world would have a reduction of 64.6 per cent in the impact of a potential disease spreading. One step further, the project identifies the 10 most important airports11, for which increasing the level of hand cleanliness (or hand washing engagement rate) only at those, the impact of the disease spreading would decrease by nine per cent to 37 per cent.
In the second phase of the project, the team aims to design and implement randomised control experiments to examine individual behavioural changes on the adoption and engagement with smart-tech hand washing systems in public places. The experiment will take place in a major international airport where Smixin stations will be set up and the behaviours of air travellers in the use of the systems will be tracked. In addition, the digital screens of the systems will be devised to display hygiene promoting messages that will give insights on how awareness can potentially change the behaviour of humans in the adoption of smart hand washing technologies.
This research can potentially shape the way policymakers design and implement strategic interventions based on promoting hand-washing in airports that will lead to the restriction of any infection within a confined geographical area at the early days of an outbreak and inhibit the expansion as a pandemic.
References
- Zhao B, Dewald C, Hennig M, Bossert J, Bauer M, Pletz MW, Jandt, KD, (2018). Microorganisms @materials surfaces in aircraft: Potential risks for public health? – A systematic review. Travel Medicine and Infectious Disease.
- Peiris JSM, Guan Y, Yuen KY, (2004). Severe acute respiratory syndrome. Nature Medicine, 10: S88–S97.
- Purdue University, (2000). Hand sanitizers no substitute for soap and water. https://www.purdue.edu/uns/html4ever/000211.Almanza.sanitizers.html
- Wayne Combs, Ph.D., Community Health Nurse, U.S. Army Public Health CommandFebruary 1, 2013 https://www.army.mil/article/95551/handwashing_the_do_it_yourself_vaccine
- Airbus, (2018). A330neo Family: Powering into the future. https://www.airbus.com/content/dam/corporate-topics/publications/backgrounders/Backgrounder-Airbus-Commercial-Aircraft-A330neo-E.pdf
- Boeing, (2016). The Airplane Bathroom That Cleans Itself. http://www.boeing.com/features/2016/03/self-clean-lavatory-03-16.page
- Bormann R, Weisshardt F, Arbeiter G, Fischer J., (2013). Autonomous dirt detection for cleaning in office environments. IEEE International Conference on Robotics and Automation, p. 1260–7.
- http://www.smixin.com/
- Nicolaides C, Cueto-Felgueroso L, Gonzalez MC, Juanes R, (2012). A metric of influential spreading during contagion dynamics through the air transportation network. PLoS ONE, 7(7): e40961.
- Nicolaides C, Cueto-Felgueroso L, Juanes R, (2013). The price of anarchy in mobility-driven contagion dynamics. J. R. Soc. Interface, 10: 20130495
- LHR, LAX, JFK, CDG, DXB, FRA, HKG, PEK, SFO, AM
Biography
Related topics
Accidents and incidents, Passenger volumes, Safety, Sustainability, Terminal operations, Tourism