AFFF hangar spill: Better outcomes, minimal environmental impacts
- Like
- Digg
- Del
- Tumblr
- VKontakte
- Buffer
- Love This
- Odnoklassniki
- Meneame
- Blogger
- Amazon
- Yahoo Mail
- Gmail
- AOL
- Newsvine
- HackerNews
- Evernote
- MySpace
- Mail.ru
- Viadeo
- Line
- Comments
- Yummly
- SMS
- Viber
- Telegram
- Subscribe
- Skype
- Facebook Messenger
- Kakao
- LiveJournal
- Yammer
- Edgar
- Fintel
- Mix
- Instapaper
- Copy Link
Posted: 30 November 2017 | Mike Willson (Fire Protection Consultant) | No comments yet
Extensive media coverage was given to a 22,000-litre spill of Aqueous Film Forming Foam (AFFF) concentrate from an aircraft hangar at Australia’s Brisbane Airport on 10 April 2017. Much of the media coverage included headlines such as “Toxic foam spill”, “Calls for AFFF ban”, and “Toxic foam begins Class Action”, ramping up community unrest and misleadingly implying a ‘toxic spill disaster’ had occurred. For International Airport Review, fire protection consultant, Mike Willson, explores the reports and outcomes following the incident.
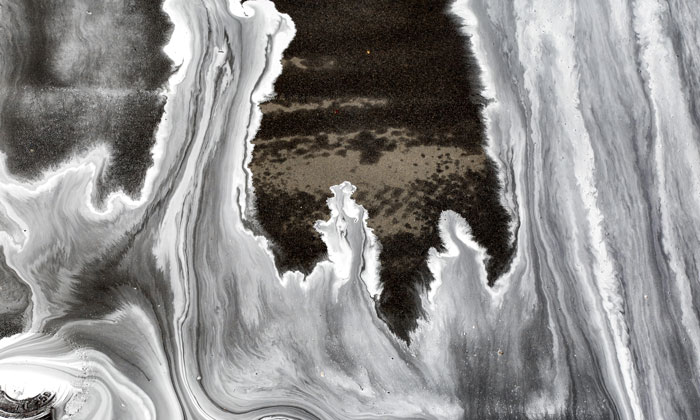
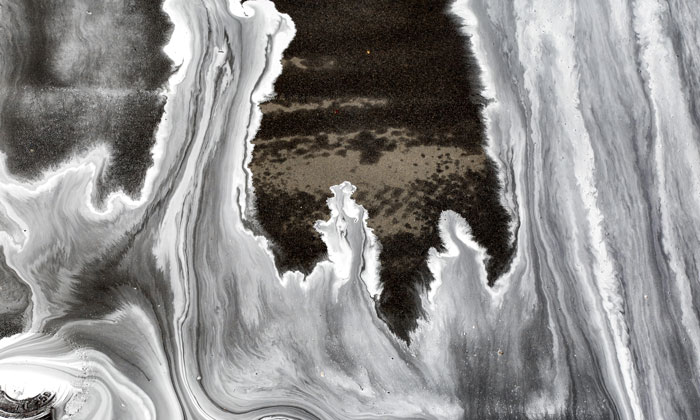
EXTENSIVE sampling data collected by Queensland’s Department of Environment and Heritage Protection (DEHP)1 from broad sampling points following the incident, commendably published on DEHP’s website, paints a different picture. The sampling results confirmed there was no significant cause for concern – either to the environment, human health, wildlife, water quality, or seafood.
PFOA hardly detected
A recent case study analysed this data (sediment, water quality, fish and crustaceans) following the spill2,3. The DEHP generally acted responsibly, with precautionary but measured community advice.
The Australian Minister for Infrastructure confirmed: “While it is known PFAS can persist for a long time, there is no consistent evidence that PFAS exposure is harmful to human health.” Queensland’s Chief Health Officer also warned the public not to eat seafood until these sampling test results were known. Queensland Health confirmed commercial and recreational fishing for bait in the investigation area was low-risk and could continue.
Most of the foam was reportedly collected and remained within the airport perimeter, but reports estimated 25 per cent (5,500 litres) of AFFF escaped offsite1, leaking into waterways and the surrounding coastal environment.
The foam was confirmed as a long-chain C8 fluorotelomer surfactant based AFFF agent1,2,4 that does not contain or breakdown to PFOS/PFHxS, which is only derived from electrofluorination. Sampling data relating to PFOS and PFHxS levels in water, sediment, fish and crustaceans had therefore probably come from another historic incident or source, unconnected with this spillage. PFOS is listed as a Persistent Organic Pollutant (POP) under the United Nation’s Stockholm Convention5, and PFHxS and PFOA are also under POP listing consideration6,7. Concerns surround long-lived PFOS/PFHxS and PFOA in sediments, water, biota and the environment, accumulating in organisms, potentially entering the human food chain and being passed into embryos and infants through mother’s milk. Areas around some Australian Defence sites show relatively high levels – particularly of PFOS contamination – after many years of firefighter training with electrofluorination based AFFFs, at these specific facilities.
A detailed analysis of the data1,2,3 reveals some surprising results.
At no time – between the day following the spill until sampling ceased on 13 June 2017 – did PFOA levels measured in water quality samples across a wide area, exceed latest stringent 2017 Food Standards Australia New Zealand (FS ANZ)8 recreational water quality levels. Specifically, these are 5.6 μg/L or parts per billion (ppb), which equates to 17 seconds over 100 years9! The highest PFOA level recorded after the incident was 1.46 ppb – far below any cause for concern.
Also, zero PFOA was recorded in any fish species sampled anywhere throughout the sampling period, following the incident.
The maximum 9 ppb PFOA occurred in two crabs close to the spill site – well below the FS ANZ trigger point of 520 ppb for crustaceans and molluscs (which include such species as crabs, shrimps, oysters, lobsters, mussels, etc.). Two crabs with lower PFOA levels were sampled a considerable distance from the spill; probably due to other causes potentially related to reclamation, consumer products, boat discharges, background levels, or from consumer products via waste water treatment outfalls. This again confirms no significant concerns for water quality, food or human health following the incident.
Furthermore, very low water quality levels of combined PFOA and all its related substances including precursors were found at 300-350 ppb levels (equivalent to just 17.5 minutes over 100 years9). This was detected the day after the spill occurred, and close to the spill location. Within seven days this had dispersed to presumed background levels, typically averaging 1.3 ppb (300 times less) until 13 June 2017. Also, 20 fish reportedly died within the airport perimeter, presumably close to the AFFF spillage. However, this should be seen in context of the preferred Fluorine Free Foam (F3) usage being encouraged by Queensland’s Management of Firefighting Foam policy10. Research confirms F3 agents are typically 10-25 times more toxic to aquatic organisms than AFFFs11-13. Had this same quantity of F3 been inadvertently spilled, it is reasonable to expect potentially 200-500 fish be killed, for which the original misleading headlines “toxic spill disaster” might have been more appropriate. Furthermore, since shellfish and crustaceans are generally more sensitive to strong detergents than fish11-14, had this been an F3 spill, or had F3 been used in a fire incident where typically three times more of a 10 times more toxic F3 agent was required for a given sized volatile fuel fire (e.g. Jet A1 or Gasoline) than AFFF3,18-20,23,26, the consequences could have been far worse. It could have potentially resulted in even larger numbers of fish deaths (600-1,500) and an accompanying substantial decline in shellfish populations, with the potential to disrupt commercial and recreational fishing across the area for some time.
This extensive sampling data1,2 confirmed there were no significant concerns for water quality, fish, crustaceans, sediments, human health, human food or the wider environment, following this AFFF spill incident.
Chasing better outcomes
In some jurisdictions, current screening and trigger levels for PFAS are incredibly low – sometimes below the level of detection, making it difficult to establish the level of precaution to take15. As we transition away from the use of long-chain fluorochemicals of concern, is this level too low to be helpful in assessing and regulating sites for PFAS contamination and future foam usage?
A 2016 South Australian EPA study16 showed: “While dolphins have high levels of PFAS, there is nothing to say that they are unhealthy because of it. The Port River and Barker Inlet dolphins are flourishing, with the last decade having the highest numbers of dolphins seen in the area since records began. Even though we can detect many chemicals in the environment, we have confidence that the environment is improving over time”. It also confirmed that: “Dolphins that reside in heavily industrialised regions – particularly the Swan River (Western Australia) and to a lesser extent the Port River (South Australia) – had some of the highest PFOS concentrations found in marine mammals worldwide. Despite this, the Port River dolphin population has increased over the last 30 years, suggesting that the population is not affected by these high concentrations”.
These Port River Dolphins had sampled mean liver PFOS concentrations of 1,986 ppb (ng/g), while the Swan River Dolphins’ mean sampled at 6,975 ppb PFOS, with 14,000 ppb in one individual16. PFOS accounted for 94 per cent and 2.4 per cent PFHxS of total PFAS recorded in Port River Dolphins, yet they remain healthy. Mean Port River inner harbour PFOS water levels were measured at 6 ppb16, significantly above current Food Standards ANZ drinking water levels of 0.07 ppb for PFOS/PFHxS and 0.56 ppb for PFOA8. However, current U.S. EPA 2016 drinking water combined PFOS and PFOA levels are set at just 0.07 ppb17. Are they realistic?
This highlights a need to establish what the indicators of significant adverse effects in humans, wildlife and the wider environment are. Shouldn’t we be using those to set concern levels for future restriction, regulation, and remediation of contaminated sites? They could also more effectively define what is – and is not – a contaminated site, to avoid everywhere being potentially labelled ‘contaminated’.
Which option to choose?
The question to address is whether we use environmentally benign short-chain C6 agents, or F3 – neither of which is ideal given that both can cause undesirable effects…as does the fire itself. Is it worthwhile to suffer slower control of fires, more noxious smoke and breakdown product releases, greater risk of incident escalation and damage, diminished life safety, and potential overflow of containment areas? Research shows each of these to be realistic and credible F3 outcomes where forceful application onto volatile fuel fires like Jet A1 and gasoline are involved18-26. F3 is acknowledged as typically at least 10 times more toxic than AFFF11-14, which is likely to kill more fish and aquatic organisms in receiving waters, especially when coupled with a larger cocktail of noxious breakdown products from the fire.
Is this potentially heavy cost burden worthwhile just to prevent usage of any persistent chemicals, even if they are not shown to be harmful?
Short-chain C6 fluorinated surfactants are typically < two per cent by weight of firefighting foam concentrate composition27, which is diluted almost 100 times to the foam solution we use. They are not shown to be bioaccumulative, or toxic, nor carcinogenic, or with developmental toxins28-36, yet they categorically provide faster, more effective, efficient and reliable fire extinguishment18-20,23-26. This delivers improved life safety, reduced escalation risk, reduced damage and community disruption, with less likelihood of containment area overflow, and are typically at least 10 times less toxic to aquatic organisms11-14 (with less volume used) than F3, should it escape into the environment. Are these really such tough considerations, given that in 2014 the UK Environment Agency recommended37 the “primary concern should be which foam is the most effective at putting out the fire. All firewater runoff and all foams present a pollution hazard”.
The UN (2009) estimated that firefighting foams typically account for five per cent of PFAS usage worldwide38. Ninety-five per cent includes all ubiquitous uses we take for granted38,39, such as stain repellent carpets, upholstery, floor waxes, hydraulic fluids, seals, cooking pans, clothing, glossy magazines, food packaging, cosmetics, pharmaceuticals etc. Each of these are disposed of through landfill (and subsequent leachate), Waste Water Treatment Plants (WWTPs), or Publicly Owned Treatment Works (POTW), which do not capture the fluorochemicals40-43 but allow substantial quantities into our waterways and oceans, every day of every year.
Even if we choose F3 for our aviation firefighting, fluorochemicals are likely to enter the environment from airport infrastructures, concrete training areas44, aircraft, supply vehicles involved in fire incident runoff, potentially overflowing containment areas. Are these inevitable F3 compromises actually worth making, or is it better to rely on environmentally more benign short-chain C6 fluorinated agent technologies?
Shouldn’t we also be addressing collection and remediation of our waste streams to extract these fluorochemicals from landfill and WWTPs, before entering our environment? That way, we would all gain the often unique benefits of more benign short-chain C6 fluorinated chemicals, while ensuring that minimal amounts can enter the receiving environment in future.
Conclusions
Contrary to some media reports, most of the contamination detected following this hangar AFFF spill appears to be largely due to historical sources of legacy contamination from PFOS-based foam agents. Extensive sampling of water quality, sediments, fish and crustaceans over a wide area from the day following the spill, has shown that Food Standards ANZ trigger levels for PFOA alone were not exceeded at any time, anywhere following this incident. Combined levels of PFOA and all its related substances peaked at 350 ppb the day following the incident at the closest monitoring site (equivalent to just 17.5 minutes over one 100 years). These combined levels had dispersed to background levels of 1.1 ppb within seven days. There were no significant concerns to human health, food, water quality, wildlife, sediments, or the wider environment following this AFFF spill incident.
Consideration should be given to focusing on restricting and regulating long-chain fluorinated foams of concern (degrading to PFOS/PFHxS and PFOA) – following the European Union’s PFOS ban in 2011 with PFOA expected in 2020 – and focus on remediation of existing significant contamination.
New generation short-chain C6 AFFFs are different; they do not break down to PFOS, PFHxS or PFOA, yet can provide equivalently high levels of firefighting performance, an essential requirement for fast control, protecting life safety, minimising damage and environmental impacts from many industrial firefighting applications, including aviation.
Greater consideration needs to be given to delivering better outcomes by addressing management, removal of major sources of historical PFOS contamination, and collection and remediation of all fluorochemical sources at WWTPs (Waste Water Treatment Plants) and landfill sites. This will prevent the ubiquitous usage of fluorochemicals we take for granted, potentially building up in our environment and causing problems in future. Focusing on the five per cent used in firefighting foams is probably not the answer. Addressing the other 95 per cent of uses as well as much of this five per cent, probably is; by collecting and remediating them all at the main WWTP and landfill sites, which are the main avenues into our environment annually. This approach could deliver improved outcomes for everyone and our environment.
Biography
References:
- Queensland (QLD) Department of Environment and Heritage Protection (DEHP), 28 Apr 2017 – Firefighting foam spillage at Brisbane Airport https://www.qld.gov.au/environment/pollution/management/incidents/brisbane-airport
- Willson M, 2017 – Case Study Part 1: Sampling Data from Qantas’ Brisbane Aircraft Hangar AFFF Spill – What does it tell us? Public Release 28 Aug 2017
- Willson M, 2017 – Case Study Part 2: Qantas’ Brisbane Aircraft Hangar AFFF Spill – Suppose a Fluorine Free Foam (F3) agent had been used instead? Public Release 28Aug 2017
- Houtz E, Higgins C, Field J, Sedlak D, 2013 – Persistence of Perfluoroalkyl Acid precursors in AFFF-Impacted Groundwater and Soil, environmental Science & Technology 2013, 47, 8187−8195 http://pubs.acs.org/doi/abs/10.1021/es4018877
- UNEP, 2009 – PFOS included in The Stockholm Convention Persistent Organic Pollutants (POP) List http://chm.pops.int/TheConvention/Overview/TextoftheConvention/tabid/2232/Default.aspx
- UNEP, 2016 – Consideration of PFOA as POP http://chm.pops.int/Convention/POPsReviewCommittee/Chemicals/tabid/243/Default.aspx
- UNEP, 2017 – Proposal for POP listing of PFHxS http://chm.pops.int/Convention/POPsReviewCommittee/Chemicals/tabid/243/Default.aspx
- Australian Government, Department of Health, 2017 – Food Standard ANZ Consolidated Report: “PerFluorinated Chemicals in Food”, April 2017 www.health.gov.au/internet/main/publishing.nsf/Content/2200FE086D480353CA2580C900817CDC/$File/Consoldiated-report-perflourianted-chemicals-food.pdf
- Parts Per Notation, 2017 – https://en.wikipedia.org/wiki/Parts-per_notation
- Queensland Department of Environment and Heritage Protection (DEHP), 2016 – Management of Firefighting Foam Policy, July 2016 www.ehp.qld.gov.au/assets/documents/regulation/firefighting-foam-policy.pdf
- Bundesamt für Wehrtechnik und Beschauffung (BWB), 1989 – German Federal Office of Defence Technology and Procurement – “Analysis of the Toxic Effects and Biological Breakdown Capabilities of Foam Extinguishing Substances in Waste Water”
- Angus Fire, 2007 – “Modern Developments in FireFighting Foams”, presented by author to broad Foam User audience at seminar in Warsaw, Poland Oct.2007
- Fire Fighting Foam Coalition, 2006 – Special factsheet on Aquatic Toxicity of firefighting Foams, www.fffc.org/images/AFFFupdatespecial.pdf
- Lenntech, 2015 – Summary of detergent impacts in freshwater ecosystems. http://www.lenntech.com/aquatic/detergents.htm#ixzz3UbwykSGh
- Nadebaum P, 2017 – Proportional Response, Industrial Fire Journal iss.100, Q3,2017 https://issuu.com/hemminggroup/docs/ifj_q3_2017
- Gaylard S, 2016 – Per and polyfluorinated alkyl substances (PFAS) in the marine environment- preliminary ecological findings, South Australia EPA, www.epa.sa.gov.au/files/12580_report_pfas_marine.pdf
- US EPA, 2016 – PFOA and PFOS Drinking Water Health Advisories – Factsheet https://www.epa.gov/sites/production/files/2016-05/documents/drinkingwaterhealthadvisories_pfoa_pfos_5_19_16.final_.1.pdf
- Schaefer T, et al, 2008 – Sealability Properties of Fluorine-Free Firefighting Foams, University of Newcastle, Australia, Fire Technology Vol 44. issue 3 pp297-309 http://novaprd-lb.newcastle.edu.au:8080/vital/access/manager/Repository/uon:4815;jsessionid=E0140D586B0467E75B68993EBC83A1CA?exact=sm_subject%3A%22vapour+suppression%22
- Jho C, 2016 – “Interactions of Fire-fighting Foam with Hydrocarbon Fuel – Some Fundamental Concepts”, Dynax Corporation (USA) presentation at Singapore Aviation Academy Foam seminar, Singapore 20 July 2016
- Castro J, 2016 – Fluorine Free Foams – Where is the Limit?, Singapore Aviation Academy and International Airport Fire Protection Association Seminar, Singapore July 2016
- Jho C, 2012 – “Flammability and degradation of fuel contaminated fluorine-free foams”, International Fire Fighter, Dec 2012, MDM publishing. www.dynaxcorp.com/resources/pdf/articles/Flammability-IFF.pdf
- “Flammable firefighting foams!” (You Tube video), 2012 – Laboratory testing to verify fuel pickup of F3 foams www.youtube.com/watch?v=IuKRU-HudSU
- “AFFF v fluorine free foam” (You Tube video), 2013 – Slower extinction and poorer burnbacks during comparative testing, www.youtube.com/watch?v=3MG2fogNfdQ
- Kleiner E, 2011 – 40 yrs of Saving Lives: C6 Fluorotelomer Surfactants and their use in Firefigthing Foams, Dynax Corporation at American Chemical Society, San Diego, USA, Mar 2016 https://ep70.eventpilotadmin.com/web/page.php?page=Home&project=ACS16spring
- Ottesen J-O, & Jönsson J-E, 2017 – AFFF v F3 Foams in Industrial Firefighting Systems – Trends, Performance, Concerns and Outlook, JOIFF Catalyst p7-8, iss3, Jul 17, http://joiff.com/wp-content/uploads/2017/07/July_2017.pdf
- Willson M, 2016 – Can Fluorine Free Foams (F3) take the fire security heat? International Airport Review, Iss 6, p31-35 – Nov 2016. http://www.firefightingfoam.com/assets/Uploads/ARTICLES-/Willson-IAR-6-2016-Can-F3-Agents-take-the-Heat.pdf
- Pabon M, Corpart J, 2002 – Fluorinated surfactants: synthesis, properties, effluent treatment, Journal of Fluorine Chemistry 114(2002) p149-156. www.sciencedirect.com/science/article/pii/S0022113902000386
- Korzeniowski S, et al, 2013 – Biodegradation, Toxicology and Biomonitoring: AFFF Fluorotelomer based Short-chain Chemistry, Reebok Conference, Bolton, UK March 2013
- Chengalis CP, Kirkpatrick JB, Radovsky A, Shinohara M, 2009a – A 90-day repeated dose oral gavage toxicity study of perfluorohexanoic acid (PFHxA) in rats (with functional observational battery and motor activity determinations). Reprod. Toxicol. 27, 342-351
- Chengalis CP, Kirkpatrick JB, Myers NR, Shinohara M, Stetson PI, Sved DW, 2009b – Comparison of the toxicokinetic behaviour of perfluorohexanoic acid (PFHxA) and nonafluorobutane -1-sulfonic acid (PFBS) in monkeys and rats. Reprod. Toxicol. 27, 400-406 www.ncbi.nlm.nih.gov/pubmed/19429410
- Loveless SE, et al, 2009 – Toxicological Evaluation of Sodium Perfluorohexanoate. Toxicology 264 (2009) 32–44. www.researchgate.net/publication/26695422_Toxicological_evaluation_of_sodium_perfluorohexanoate
- Iwai H, Shinohara M, Kirkpatrick J, Klaunig JE, 2011 – A 24-Month Combined Chronic Toxicity/Carcinogenicity Study of Perfuorohexanoic Acid (PFHxA) in Rats, Poster Session, Society of Toxicologic Pathology, June 2011 www.daikin.co.jp/chm/products/pdf/pfoa/PFHxA/16_PFHxA_E.pdf
- Russell, Nilsson, Buck, 2013 – Elimination Kinetics of PerFlouroHexanoic Acid in Humans and comparison with mouse, rat and monkey, Chemosphere, Sep2013 ISSN 1879-1298 www.biomedsearch.com/nih/Elimination-kinetics-perfluorohexanoic-acid-in/24050716.html
- Serex T, et al, 2008 – Evaluation of Biopersistence Potential Among Classes of Polyfluorinated Chemicals using a Mammalian Screening Method. SOT 2008 Poster #958
- Hoke, et al, 2015 – Aquatic hazard, bioaccumulation and screening risk assessment for 6:2 Fluorotelomer sulfonate, Chemosphere 128(2015) pp258-265 www.ncbi.nlm.nih.gov/pubmed/25725394
- Olsen G, et al, 2007 – Evaluation of the Half-life (T1/2) of Elimination of Perfluorooctanesulfonate (PFOS), Perfluorohexanesulfonate (PFHxS) and Perfluorooctanoate (PFOA) from Human Serum. www.chem.utoronto.ca/symposium/fluoros/pdfs/TOX017Olsen.pdf
- Gable M, 2014 – “Firefighting foams: fluorine vs non-fluorine”, UK Environment Agency, Fire Times, Aug-Sep 2014
- UNIDO, 2009 – PerFluoroOctane Sulfonate (PFOS) Production and Use: Past and Current Evidence www.unido.org/fileadmin/user_media/Services/Environmental_Management/Stockholm_Convention/POPs/DC_Perfluorooctane%20Sulfonate%20Report.PDF
- Guo (US EPA), 2009 – PerFluoroCarboxylic Acid content in 116 Articles of Commerce, http://nepis.epa.gov/Exe/ZyPDF.cgi/P100EA62.PDF?Dockey=P100EA62.PDF
- Ahrens, et al, 2009 – Polyfluorinated compounds in waste water treatment plant effluents and surface waters along the River Elbe, Germany www.researchgate.net/publication/26271870_Polyfluorinated_compounds_in_waste_water_treatment_plant_effluents_and_surface_waters_along_the_River_Elbe_Germany
- Murakami M, et al, 2009 – Evaluation of Wastewater and Street Run-off as Sources of Perfluorinated Surfactants (PFSs). www.ncbi.nlm.nih.gov/pubmed/19054542
- Pistocci A and Loos R, 2009 – A map of European Emissions and Concentrations of PFOS and PFOA, Environ. Sci. Technol. 2009 43, 9237–9244 http://pubs.acs.org/doi/abs/10.1021/es901246d
- Gallen, Baduel, Mueller, et al, 2014 – Spatio-temporal assessment of perfluorinated compounds in the Brisbane River system, Australia: Impact of a major flood event www.researchgate.net/publication/260641521_Spatio-temporal_assessment_of_perfluorinated_compounds_in_the_Brisbane_River_system_Australia_Impact_of_a_major_flood_event
- Baduel C, et al, 2015 – Perfluoroalkyl Substances in a Firefighting Training Ground (FTG), Distribution and Potential Future Release, www.researchgate.net/profile/Christine_Baduel/publication/276151390_Perfluoroalkyl_substances_in_a_firefighting_training_ground_FTG_distribution_and_potential_future_release/links/55e7b9a708ae21d099c15634.pdf?origin=publication_detail&ev=pub_int_prw_xdl&msrp=bbVZSR_iYRA8qxCUd8zNXPQ4qvb04fqJ1JZ47Lj1PYz6XuKSp3zr-15tIFxHMojlH7f4BJ9xuz8fjfoEb6ZH-w.K586zRpGT3xjnS63DMN1y-bd5dxAhe3Tv0A5g4ycRffvHZJQHwEZE-6yvhDuh_iewb8IjTuNFv4wqsqvI0JaJg.42lkF_qtvbGO2lB9nqNtCR7TxR5vR1nMXro3r-7chGOfmWTmxrBiAIG7Vi8lA7pUsfg5eHFCOxVGNDqevUvH0w
- Anyone interested in reviewing this case study further, should please contact the author at [email protected] for a free copy.
Issue
Related topics
Accidents and incidents, Aircraft rescue and firefighting (ARFF), Safety, Sustainability
Related airports
Related organisations
Queensland’s Department of Environment and Heritage Protection (DEHP)