The secrets to faster boarding
- Like
- Digg
- Del
- Tumblr
- VKontakte
- Buffer
- Love This
- Odnoklassniki
- Meneame
- Blogger
- Amazon
- Yahoo Mail
- Gmail
- AOL
- Newsvine
- HackerNews
- Evernote
- MySpace
- Mail.ru
- Viadeo
- Line
- Comments
- Yummly
- SMS
- Viber
- Telegram
- Subscribe
- Skype
- Facebook Messenger
- Kakao
- LiveJournal
- Yammer
- Edgar
- Fintel
- Mix
- Instapaper
- Copy Link
Posted: 16 September 2005 | Kai Nagel, Professor of Transport Systems Planning and transport telematics, Technical University of Berlin and Pieric Ferrari, ETH Zurich | No comments yet
The latest research into the way passengers behave when boarding aircraft reveals some surprising results, and prompts reflection on present boarding practices.
The latest research into the way passengers behave when boarding aircraft reveals some surprising results, and prompts reflection on present boarding practices.
It goes without saying that airplanes only make money when they are in the air. Considering today’s tough competition and the pricing pressures in the passenger carrying business, this insight becomes especially important. On one hand, airplanes must work at full capacity and idle times should be avoided. On the other hand, punctuality as an important contribution to the passenger’s service has to be ensured. However, masses of passengers waiting for hours in overcrowded airport facilities during the holiday season indicate that this is not happening.
The reduction of idle time on the ground will lead to improved airplane utilisation and more flexible time management. How, then, can these idle times be reduced?
Turn-around times
Optimisations of turn-around times can start at any point between arrival and departure. The deplanation process, aircraft cleaning, refuelling, cargo (un-)loading and passenger boarding are the main elements of the turn-around time. Passenger boarding is the part that takes the longest time and because cargo loading can be done at the same time it is also the most important one.
Plenty of different solutions have been proposed in the past to reduce passenger boarding times. Their common goal is to get all passengers seated in their assigned seat in a fast and efficient way. Yet the airplane interior does its best to prevent fast passenger movement. Another major obstruction is hand luggage, which when carried on board adds to the congestion in the narrow aisles, preventing travellers from passing.
A current popular solution is to divide passengers into so-called boarding groups that enter the airplane sequentially and are intended to avoid congestion. The call-off is realised by announcing the rows through speakers or by means of lamps corresponding to a color-coded boarding card. But do these devices really do their job? And what would a good boarding policy look like?
One possible way to answer these questions would be to conduct large-scale experiments with real passengers and real planes using different boarding policies. However, it is apparent that such a project would generate high costs, while permitting only few runs in an acceptable time frame. A more clever approach is the use of computer simulations in the first stage. Passengers’ behaviour can be reproduced by software that allows the close analysis of the boarding process.
Software simulating behaviour
To simulate the boarding process inside the aircraft we use a microscopic cell-based simulation, which means that each individual is represented in a grid as an occupied cell that moves according to specified rules which reproduce behaviour. All conditions that can influence the simulation result are integrated into models and formulated mathematically. These conditions can, of course, be easily described in words.
The aircraft model defines the dimensions of the airplane as well as the interior layout, i.e. the spacing between seats. For our considerations we use a typical short haul configuration; an airplane consisting of 123 seats that are distributed over 23 rows. The walking speeds of passengers and restrictions on movement such as obstructions that passengers cannot pass in the aisle are included in the passenger model. The seating model also contains movement decisions while seating. For example, consider that passengers occupying a middle seat have to get up for people with window seats. Lastly is the bin occupancy model, where carry-on luggage is taken into account. To every passenger, certain pieces of luggage are assigned in compliance with a predefined distribution (e.g. 60 per cent of passengers are carrying only one piece). In the simulation it takes longer for a traveller to store more pieces of luggage.
Boarding patterns
The simulation models explained above were then applied to different boarding strategies. A boarding strategy determines how boarding groups, which enter the airplane separately, are arranged over the seats inside the aircraft. The result is patterns such as those in figure 1. It should also be remembered that the simulation environment we built not only enables us to take a closer look at existing solutions but also allows us to easily implement every strategy imaginable.
An often-used boarding scheme is to seat passengers from the back to the front in different quantities of blocks. Groups are announced as ‘rows 10 to 15’, for example. In addition, these blocks can be divided by the aisle, meaning that passengers sitting on the right and the left will board separately. Another approach is to form boarding groups according to seat letters; to first let people with window seats in, then those assigned to middle seats and finally passengers with aisle seats. This is intuitively a good idea, as this way prevents passengers having to vacate their seat for their neighbour.
We also implemented strategies where each boarding group is formed by a single passenger only. When the boarding sequence is determined completely the simulations confirm that these strategies have the best average behaviour. However, this approach is too complicated for real-world use.
All of the boarding schemes mentioned above can be alternated by changing the sequence of the boarding group. Of course, by far the simplest method is to use no boarding group at all. This corresponds in our simulation to one single boarding group; people enter the aircraft completely unordered. We also implemented outside-in-back-to-front-strategies and simplified them by merging groups, this in turn leading to ‘pyramid-strategies’, called after their shapes which become apparent in a top view while passengers are boarding.
Ranking of strategies
For airlines introducing new boarding strategies not only is the average boarding time of interest but so is the possibility of very bad boarding times. Taking this into consideration, we did not rate boarding strategies according to the average boarding time, but instead looked at the average worst case of the boarding duration over 50 replications. A plausible interpretation of our measure is that approximately 95 per cent of all boarding events are faster than our number. All of our results are given in simulation time steps that behave proportional to absolute boarding time.
In general a strategy will show good performance when it reduces conflicts. Conflicts are defined as all constellations in which passengers can’t move forward or sit down because of others. A good strategy must also show good performance, must work whether passengers arrive early or late and should be suitable for different airplanes with varying interior layout. As airplanes are not always full, boarding strategies should be efficient with smaller occupancies. However, boarding using the same scheme with fewer passengers will, on average, always be faster than with a full plane. As long as the flight schedule is not adjusted to the expected load, there is little need to test reduced occupancies.
The simulation in action
We implemented approximately 60 boarding strategies and benchmarked them for robustness against disturbances.
If passengers are divided into boarding groups, it will often occur that some arrive early or late. Results show that there is no significant difference between the two possibilities. In other words, if 20 per cent of the passengers are off-time, half of them early and half of them late, then the effect of the disturbances can be reduced to 10 per cent if all early boarding attempts are rejected at the ticket reader system.
We tested how much the percentage of passengers not arriving in time will influence the quality of the boarding strategies. In figure 2 the resulting performance-profile can be seen for 0 per cent, 20 per cent, 40 per cent and 80 per cent of passengers arriving off time. The simulations show that even with disturbances, block-strategies (back-to-front-boarding) perform worse than plain random boarding (no boarding strategy) indicated by the red-coloured part in figure 2.
More importantly, this corresponds to a troubling result, that when more passengers do not follow their boarding groups these strategies improve. Since this contradicts common-sense reasoning, we will clarify this point. Boarding back-to-front essentially causes a great deal of conflict-causing loading activity in the current boarding block – while there is no loading in other parts of the airplane. In this situation, passengers boarding at times when they are not called leads to a situation where loading occurs in the areas of the aircraft where there is little current activity, thus increasing the amount of loading that can occur simultaneously. We therefore concluded that there is no justification for airlines to use block-boarding from the viewpoint of reducing boarding time.
The boarding time of the row-strategy, which fills the airplane row-wise from back to front, exceeds the results of all other strategies. It maximizes the number of conflicts in the current block, resulting in very bad performance. A strategy of alternating rows (skipping rows) helps reduce the number of passengers that are waiting because the passenger in front fits in between the busy rows, relieving the delay. Please refer to the green highlighted parts in figure 2. Obviously, such strategies are highly dependent on airplane layout and thus not recommended.
Strategies that fill the airplane by seat letters from window to aisle show good performance and are also robust against disturbances (represented by the dark-blue coloured part in figure 2). They are an excellent proposal, although they can be expected to split passengers travelling in groups such as families and are therefore unpopular in practice.
The lowest peak in figure 2 corresponds to the case where the sequence of boarding passengers is determined by the individual. Every boarding group consists of only one member, so to speak, and interferences between passengers can be minimized by lining them up in the right order (light-blue shaded part of figure). Owing to the exorbitant number of boarding groups, these types of strategies are also impractical and unusable.
Seatgroup-strategies attempt to reduce the amount of boarding groups building by using small groups of passengers instead of single passengers. As expected, this strategy also shows good performance and acceptable robustness, but still requires too many boarding groups.
For a further reduction of boarding groups, merging boarding groups diagonally leads to pyramid strategies. These retain the good efficiency and promise robust behaviour against airplane layout changes and other disturbances. These strategies are also recommended.
Conclusion
The simulation confirms the expected result; that when using a suitable strategy the process of boarding an aircraft can be improved considerably (refer to figure 3). Even simple boarding strategies save an average of more than 20 per cent of boarding time.
However, the often-used block-strategies are inefficient and can be seen to delay the boarding process compared to random boarding. In fact travellers not obeying the boarding calls will improve those strategies. A further interesting observation is that the amount of carry-on luggage has a strong influence on boarding times and that restrictions will also lead to faster boarding.
The strategies which show greater sensitivity to airplane interior layout changes are a good choice. Recommended strategies are letter-, seatgroup- and pyramid-strategies, which all show good efficiency and robustness. It should be remembered though, that all of these proposed schemes are only applicable in combination with call-off systems and direct access (boarding through a finger dock).
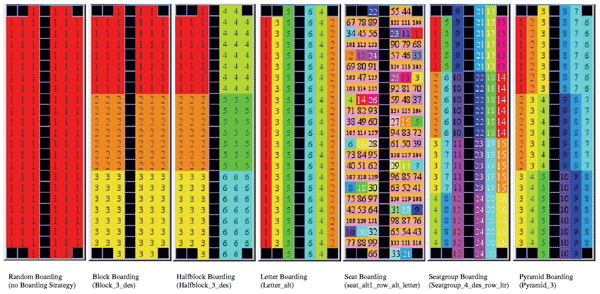
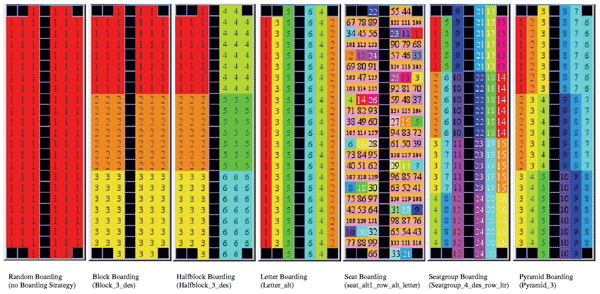
Figure 1: Graphical representation of boarding strategies
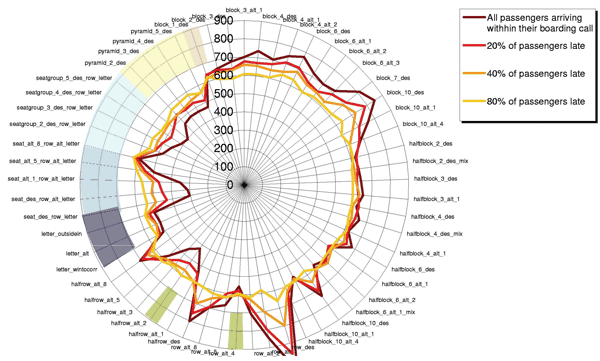
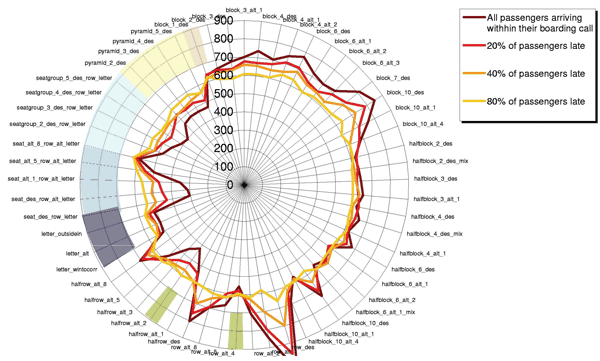
Figure 2: Performance profile of boarding strategies for different percentages of disturbances. Values are given in valuation timesteps
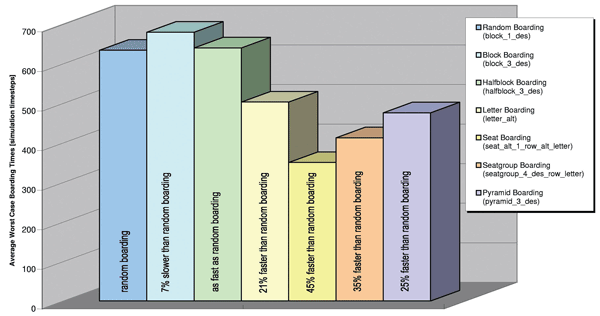
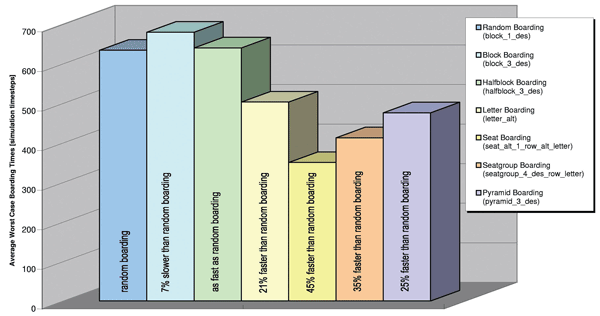
Figure 3: Comparison of chosen boarding strategies
Pieric FerrariP
ieric Ferrari studied Computer Science at the Swiss Federal Institute and gained a Masters Degree in Computer Science in 2004. His work on passenger simulation in airplanes for the Institute for Scientific Computing, ETH Zurich 2003-2005 resulted in a paper presented at the Transportation Research Board Annual Meeting 2005.
Kai Nagel
Kai Nagel has a Ph.D. in Computer Science awarded from the University of Cologne in Germany. His thesis was about fast microscopic traffic simulations. He is currently a Professor for Transport Systems planning and transport telematics at the Technical University of Berlin, a position he has held since April 2004.