Foreign Object Detection: Smart airport pavement health monitoring
- Like
- Digg
- Del
- Tumblr
- VKontakte
- Buffer
- Love This
- Odnoklassniki
- Meneame
- Blogger
- Amazon
- Yahoo Mail
- Gmail
- AOL
- Newsvine
- HackerNews
- Evernote
- MySpace
- Mail.ru
- Viadeo
- Line
- Comments
- Yummly
- SMS
- Viber
- Telegram
- Subscribe
- Skype
- Facebook Messenger
- Kakao
- LiveJournal
- Yammer
- Edgar
- Fintel
- Mix
- Instapaper
- Copy Link
Posted: 2 June 2015 | Halil Ceylan, Kasthurirangan Gopalakrishnan and Sunghwan Kim of Iowa State University
In light of several incidents of runway cracking and blowup in the last few years, Halil Ceylan, Kasthurirangan Gopalakrishnan and Sunghwan Kim of Iowa State University’s Program for Sustainable Pavement Engineering and Research (PROSPER) review ‘smart’ technologies that can improve the efficiency and effectiveness of surface monitoring…
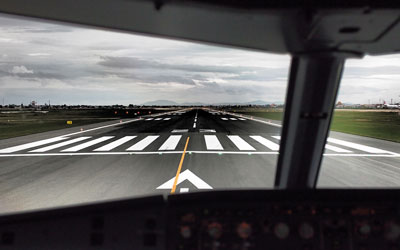
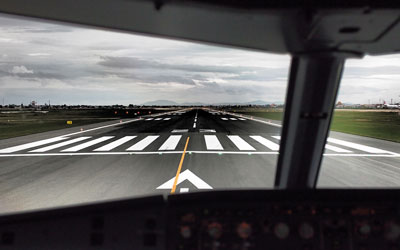
In 2014, a $225 million concrete runway pavement that was added to the Detroit Metropolitan Airport in 2001, expected to last for 30 years, began manifesting cracks and flaws just three years after it was opened. The problem seemed to have resulted from alkali-silica reaction (ASR), a phenomenon that causes concrete exposed to moisture to crack due to the reaction between aggregates containing certain forms of silica and with alkali hydroxide in concrete. Detroit Metropolitan Airport is now in the early stages of planning a reconstruction of the runway as loose pieces of concrete dislodged from the deteriorating concrete runway pose a serious foreign object debris (FOD) related safety hazard.
Similarly, the Central Region Airport Certification Bulletin reported an airport runway pavement blowup failure at Ankeny Regional Airport in Iowa during summer 2011 (see Figure 1). Excessive hot weather and the associated heat wave reportedly caused the pavement to blow up and buckle. As a result, a Raytheon Premier One jet hit the blow up spot during take-off and damaged its landing gear.
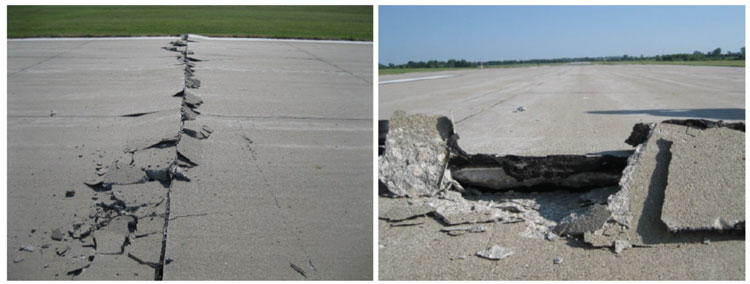
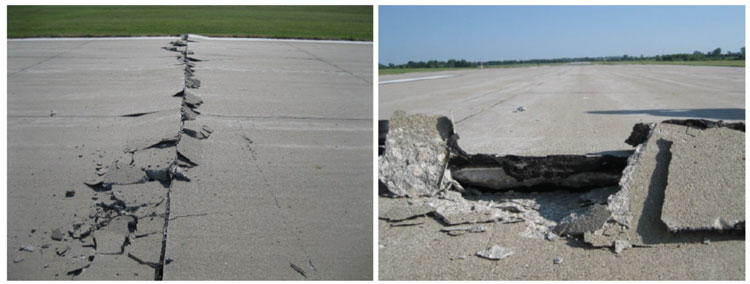
Figure 1: Concrete pavement blowup of the runway at Ankeny Regional Airport due to slab expansion resulting from excessive heat. Photo Courtesy of Snyder & Associates, Inc/Polk County Aviation Authority
These are just a few examples of how critical it is to monitor the health of airport pavements and the need for having an early warning of excessive conditions or for impending failure. Realistic characterisation of pavement layer properties and responses under in-situ field conditions is critical for accurate airport pavement life predictions, planning pavement management activities and for calibration and validation of mechanistic-based pavement response prediction models.
It is forecasted by the Federal Aviation Administration (FAA) that the annual US air industry will grow from 731 million passengers in 2011 to 1.2 billion in 2032. As per the needs of the FAA’s Next Generation Air Transportation System (NextGen), this necessitates faster and more efficient pavement condition evaluation (i.e. minimal closure of runways and taxiways) and delivery of comprehensive and rational report summaries to airport managers who are in charge of pavement maintenance and repair decisions.
Is it possible to gain a better understanding of what’s going on with your runway pavement by closely monitoring and reporting on the condition of pavements subjected to repeated traffic loads and/or environmental/climatic forces, without having to shut down the runway/taxiway?
The answer is yes, according to recent research.
Smart sensors for efficient health monitoring
Recent advancements in smart sensing technologies include: micro-electro-mechanical sensor (MEMS), nano-electro-mechanical sensor (NEMS), and fibre optic sensor (FOS) technologies. Combined with wireless sensor networks and efficient energy scavenging paradigms, they provide opportunities for long-term, continuous, real time response measurement and health monitoring of airport pavement systems.
Traditional sensing and condition monitoring approaches utilising wired sensors have been used to track pavement responses under environmental and traffic loads, including temperature, moisture, strain, stress, deflection, etc. However, over the past twenty years, studies and projects involving traditional wired sensors have reported on several disadvantages with the use of these systems, such as the need for high array density, wire damage, high installation cost and time, and low survivability of wired sensors for long-term operation, etc.
The concept behind making a structural system ‘smart’ is to embed sensing/actuation technologies within the system to report on the structure’s response in real time to external forces, thus providing a better insight into the structural behavior and bearing capacity on a continual basis2. In short, smart health monitoring systems should enable structures to be capable of real time, continuous sensing of both external and internal condition changes and responding to these changes to improve performance without human intervention.
Previous highlighted studies on MEMS/NEMS sensors for pavement monitoring include ‘Smart Pebbles’3, ‘Smart Dust’4,5, MEMS sensors capable of measuring temperature and moisture inside concrete6 and more recently, the ‘smart pavement monitoring system’7 and ‘self-sustaining damage detection sensor’8. Based on a comprehensive review of literature and vendor survey, we have synthesised the latest information available, as of 2013, on off-the-shelf MEMS devices, as well as research prototypes, for bridge, pavement and traffic applications9.
Similarly, FOS is another promising smart sensing technology for airport pavement health monitoring. A fibre optic sensor can either monitor environmental conditions or transmit data using fibre optic communication that modulates a light beam within the fibre. In general, fibre optic sensors have a small size and weight and can be used in explosive and corrosive environments. They can also be used to provide distributed sensing along the optical fibre. Theoretically, hundreds of locations along a fibre just one metre long can be measured. Furthermore, fibre optic sensors can be used to measure strain, temperature, humidity, pH, etc.
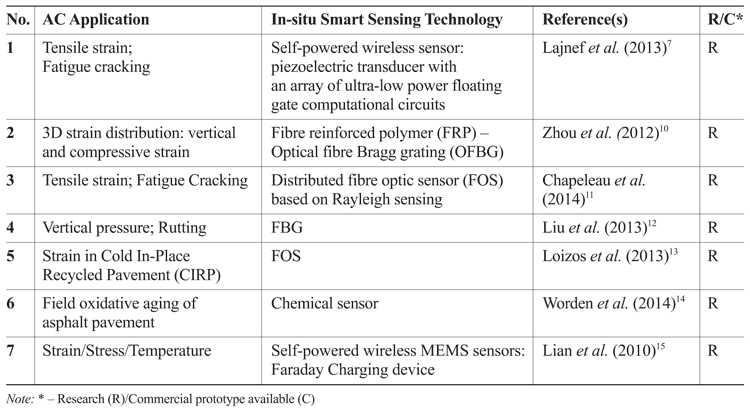
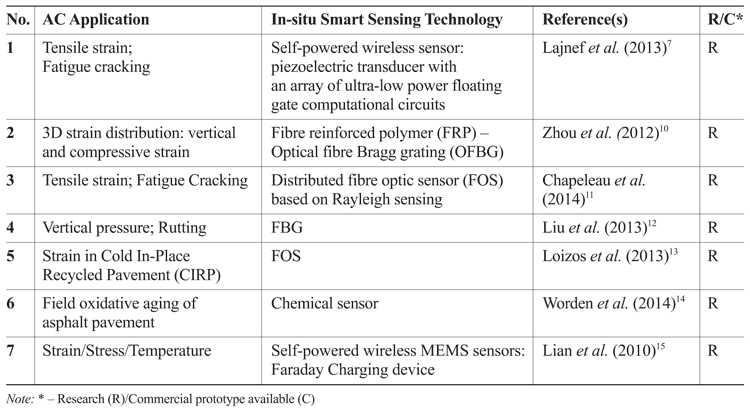
Table 1: Summary of potential recent smart sensing-based Asphalt Concrete (AC) applications with implication for airport pavement health monitoring.
Table 1 and Table 2 summarise some areas of highway infrastructure condition monitoring where the application of in-situ/embedded smart sensing (MEMS, NEMS, FOS, etc.) technology is being actively researched or have been identified for future research, with potential implications for airport pavement health monitoring.
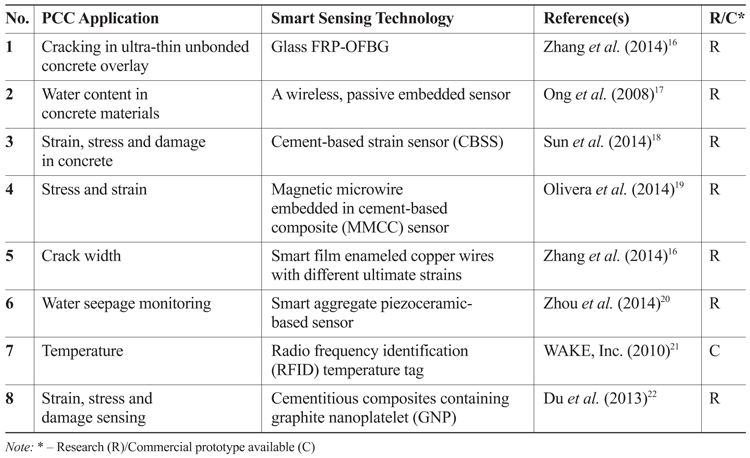
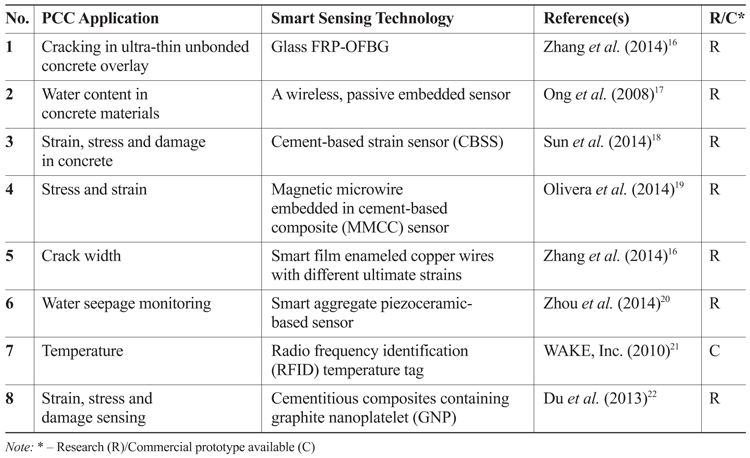
Table 2: Summary of potential recent smart sensing-based Portland Cement Concrete (PCC) applications with implications for airport pavement health monitoring.
Looking to the future: Self-sensing airport pavements
The use of embedded or surface mounted micro/nano sensors and devices is definitely a step forward in the in-situ characterisation of airport pavement responses and properties. Yet, these systems suffer from drawbacks, including low survival rate, incompatibility with the structural systems, high cost, etc. To overcome these limitations, researchers have put forward the idea of self-sensing or multifunctional structural systems where the structural system itself has the sensing capability apart from its main load bearing function. For instance, self-sensing (piezo-resistive) cement-based materials, engineered by incorporating electrically conductive fillers into the matrix, have the potential to monitor strain, stress, or cracking in themselves (through changes in electrical properties) while maintaining adequate mechanical properties.
Apart from self-sensing capabilities, multifunctional infrastructure systems can be nano-engineered to derive other non-structural functions such as self-healing, self-heating, and self-cleaning capabilities. Researchers at Iowa State University (ISU) are currently investigating self-heating airport pavements through a FAA/PEGASAS sponsored research grant. A 3D visualisation schematic and artistic rendition of a futuristic multifunctional airport runway is illustrated in Figure 2.
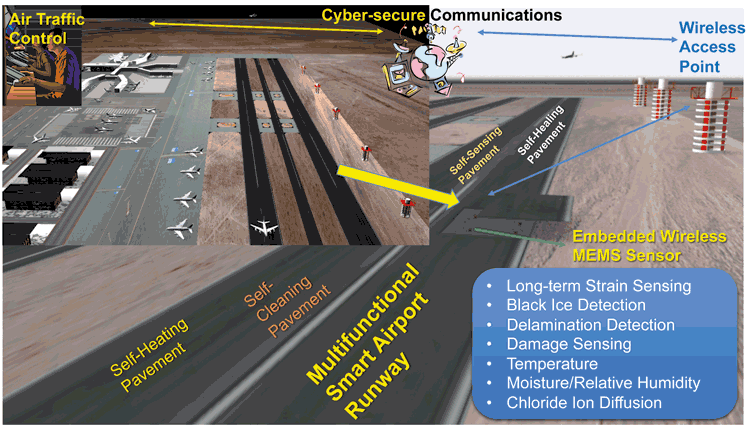
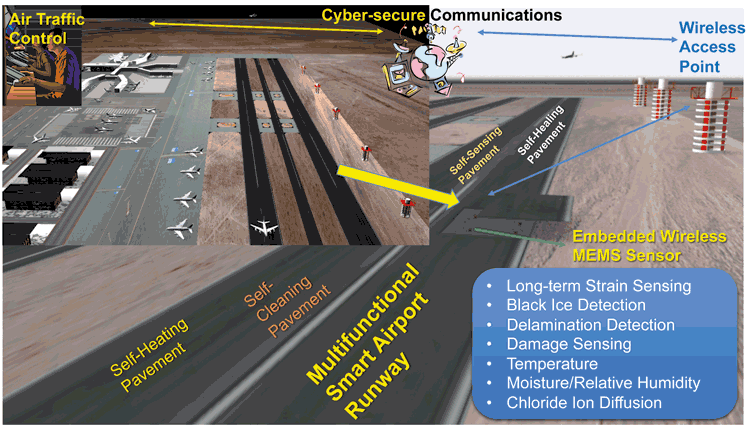
Figure 2: Artistic rendition of a futuristic multifunctional smart airport runway
This project proposes a three-pronged approach to be run in parallel, which will investigate the efficacy and cost effectiveness of new heated pavement technologies. Task 1-A will investigate the relative energy and monetary needs required to remove snow from a slab by conducting an energy and financial viability analyses. Task 1-B will involve a hybrid approach combining electrically conductive concrete with lotus leaf-inspired super-hydrophobic surfaces. Task 1-C will investigate the application of nano-coatings of low temperature phase change materials with the intent of preventing ice and slush formation.
In carrying out these tasks, we hope to better understand the costs of installing and running such systems, as well as improve operational efficiency and safety at airports and reduce the burden of snow and ice removal.
References
- Lawrence, ED (2015). Flawed Concrete Found on Detroit Metro Airport Runway: Pavement was to last until 2031, but now poses risks to safety. Detroit Free Press. Available at: http://www.freep.com/story/news/local/michigan/wayne/2015/01/04/airport-needs-rebuild-million-runway/21236323/
- Lynch, JP (2002). Decentralization of Wireless Monitoring and Control Technologies for Smart Civil Structures. Technical Report No.140, John A Blume Earthquake Engineering Center. Stanford, CA.
- Watters, DG et al. (2003). “Smart pebble: wireless sensors for structural health monitoring of bridge decks.” Proceedings of the SPIE, Smart Structures and Materials 2003: Smart Systems and Nondestructive Evaluation for Civil Infrastructures, 5057, pp. 20-28.
- Pei, JS et al. (2007). Monitoring Pavement Condition Using “Smart Dust” under Surge Time Synchronization. Proceedings of the SPIE, Sensors and Smart Structures Technologies for Civil, Mechanical, and Aerospace Systems, Vol.6529, pp. 173–182.
- Pei, JS et al. (2009). “An Experimental Investigation of Applying Mica2 Motes in Pavement Condition Monitoring”. Journal of Intelligent Material Systems and Structures. Vol.20, pp. 63-85.
- Norris, A, Saafi, M & Romine, P (2008). “Temperature and moisture monitoring in concrete structures using embedded nanotechnology/microelectromechanical systems (MEMS) sensors”. Construction and Building Materials, Vol. 22, No. 2, pp. 111-120.
- Lajnef, N, Chatti, K, Chakrabartty, S, Rhimi, M, & Sarkar, P (2013). Smart Pavement Monitoring System. FHWA Publication No. FHWA-HRT-12-072, Michigan State University, MI.
- Kuang, KSC (2014). “Development of a Wireless, Self-Sustaining Damage Detection Sensor System Based on Chemiluminescence for Structural Health Monitoring”. Proceedings of SPIE, Smart Sensor Phenomena, Technology, Networks, and Systems Integration, Vol. 9062.
- Ceylan, H et al. (2013). “Highway Infrastructure Health Monitoring Using Micro-Electromechanical Sensors and Systems (MEMS)”. Journal of Civil Engineering and Management, Vol. 19, Issue sup1, S188–S201.
- Zhou, Z et al. (2012). “Optical fiber Bragg grating sensor assembly for 3D strain monitoring and its case study in highway pavement”. Mechanical Systems and Signal Processing, Vol. 28, pp. 36-49.
- Chapeleau, X, Blanc, J, Hornych, P, Gautier, JL & Carroget, J. Use of Distributed Fiber Optic Sensors to Detect Damage in a Pavement. Presented at: Le Cam, Vincent and Mevel, Laurent and Schoefs, Franck. EWSHM – 7th European Workshop on Structural Health Monitoring, July 2014, Nantes, France
- Liu, H, Liang, D, Han, X, and Wang, X (2013). “Design of a sensor for asphalt pavement pressure field measurements”. Proceedings of SPIE, International Conference on Optics in Precision Engineering and Nanotechnology (icOPEN2013), Vol. 8769.
- Loizos, A, Plati, C & Papavasilou, V (2013). “Fiber optic sensors for assessing strains in cold in-place recycled pavements”. International Journal of Pavement Engineering, Vol 14(2), pp 125-133.
- Worden, RM, Lajnef, N, & Chatti, K (2014). “Feasibility of developing a chemical sensor for asphalt aging”. Contract No. DTRT13-G-UTC44, Michigan State University, East Lansing. Available at: http://trid.trb.org/view/2014/P/1330246.
- Lian, K (2010). “Developing embedded wireless strain/stress/temperature sensor platform for highway applications”. Technical Report No. NCHRP IDEA Project 129, Highway IDEA Program, Louisiana State University, Baton Rouge.
- Zhang, B et al. (2014). “Crack width monitoring of concrete structures based on smart film”. Smart Materials and Structures, Vol. 23, No. 4.
- Ong, JB et al. (2008). “A wireless, passive embedded sensor for real-time monitoring of water content in civil engineering materials. IEEE Sensors Journal, Vol. 8, No. 12, pp. 2053-2058.
- Sun, M, Liew, RJY, Zhang, M, & Li, W (2014). “Development of cement-based strain sensor for health monitoring of ultra high strength concrete”. Construction and Building Materials, Vol. 65, pp. 630-637.
- Olivera, J et al. (2014). “An embedded stress sensor for concrete SHM based on amorphous ferromagnetic microwires”. Sensors, Vol. 14, No. 11, pp. 19963-19978.
- Zhou, D et al. (2014). “Feasibility of water seepage monitoring in concrete with embedded smart aggregates by P-wave travel time measurement”. Smart Materials and Structures, Vol. 23, No. 6.
- WAKE, Inc. (2010). The HARD TRACK System. Available at http://www.wakeinc.com/hardtrack.html (Accessed Oct 19, 2014).
- Du, H, Quek, ST, & Dai Pang, S (2013). “Smart multifunctional cement mortar containing graphite nanoplatelet”. Proceedings of SPIE, Sensors and Smart Structures Technologies for Civil, Mechanical, and Aerospace Systems, Vol. 8692.
Biographies
Halil Ceylan is an Associate Professor in the Department of Civil, Construction and Environmental Engineering (CCEE), and Director of the Program for Sustainable Pavement Engineering and Research (PROSPER) at the Institute for Transportation (InTrans) at Iowa State University (ISU). He received his PhD in Civil Engineering in 2002 from the University of Illinois at Urbana-Champaign, USA, and he has been with ISU since then.
Kasthurirangan “Rangan” Gopalakrishnan is a Research Associate Professor in the CCEE, and Associate Director of PROSPER at the InTrans at ISU. He received his PhD in Civil Engineering in 2004 from the University of Illinois at Urbana-Champaign, USA.
Sunghwan Kim is a Research Scientist and an Associate Director of PROSPER at the InTrans at ISU. He received his PhD in Civil Engineering in 2008 from ISU, and he has been with ISU since then.
Issue
Related topics
Foreign object detection (FOD), Runways and pavements, Safety